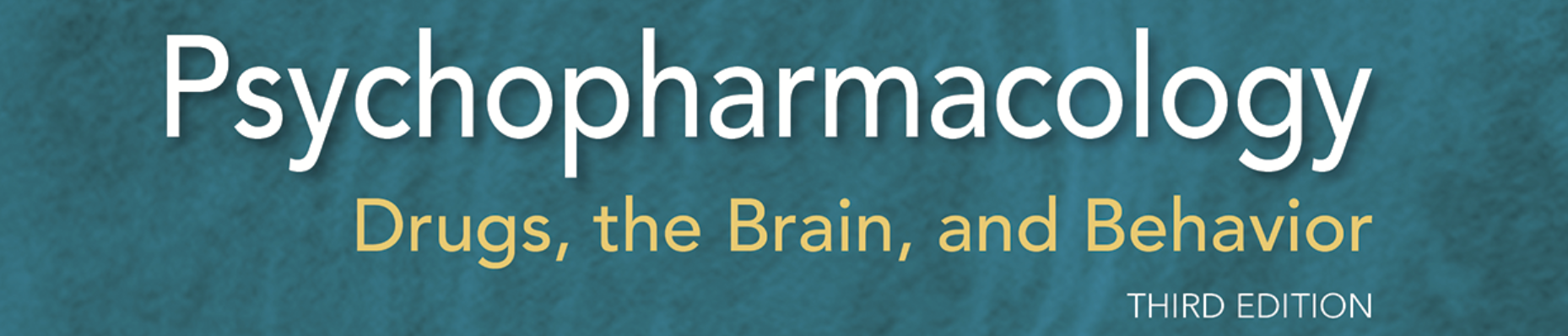
Psychopharmacology 3e Web Box 8.2 - Pharmacology in Action: What Is the Endogenous Ligand for the Benzodiazepine Receptor?
Pharmacologists sometimes work in seemingly strange ways. Traditionally, you identify a new neurotransmitter and then search for the receptors for that transmitter. Sometimes, however, a receptor is discovered that is not responsive to any known neurotransmitter, hormone, or other signaling agent. Such a receptor is termed an orphan receptor. If you discover one of these, then you need to work “backwards,” in that you must try to find the neurotransmitter that activates your orphan receptor.
What if you discover a new receptor by virtue of its sensitivity to either a plant-derived substance or a synthetic drug? As an example, the existence of opioid receptors was hypothesized and subsequently proven based on the behavioral and physiological effects of morphine, a substance found only in the opium poppy. The same reasoning later led to the discovery of cannabinoid receptors, which are activated by the psychoactive substances present in cannabis plants. In this chapter, we have seen that there are BDZ receptors in the brain that are activated by synthetic drugs. Is it possible that the brain possesses receptors whose sole function is to respond to either plant-derived or synthetic compounds? Evolutionary theory argues against such a possibility. Consequently, we need to consider the possibility that these receptors arose in the course of evolutionary history to serve signaling molecules that are made in our own brains as well as in the brains of animals. We use the term endogenous ligand to designate the body’s own signaling molecule(s) used to activate a receptor that was initially discovered because of its sensitivity to plant-derived or synthetic substances. Endogenous means “occurring within the body,” and ligand refers to any compound that binds to a receptor (see Chapter 1).
Over time, endogenous ligands have been discovered for some of these novel receptors but not others. Two well-known success stories involve the discovery of endorphins and enkephalins as endogenous ligands for opioid receptors (see Chapter 11) and the later discovery of anandamide and other so-called endocannabinoids that function as endogenous ligands for cannabinoid receptors (see Chapter 14). A receptor for which the endogenous ligand has still not been conclusively identified is the phencyclidine (PCP) receptor (as mentioned earlier, this is a site within the NMDA receptor channel). Until several years ago, the same could be said of the BDZ receptor; however, as we discuss below, researchers have now reported reasonably strong evidence for at least one endogenous ligand for that receptor.
The discovery of a BDZ binding site on the GABAA receptor more than 40 years ago prompted the eminent pharmacologist Leslie Iversen to hypothesize the existence of an anxiety-modulating substance within the brain (Iversen, 1977). During the intervening period, researchers have proposed several different candidate molecules that might serve this function (reviewed by Farzampour et al., 2015). By far, the major contender has been a large peptide (consisting of 86 amino acids) known as diazepam binding inhibitor (DBI) along with two smaller peptides that are derived from DBI. These smaller molecules are named octadecaneuropeptide [ODN], an 18 amino acid peptide consisting of DBI amino acid residues 33–50, and triakontatetraneuropeptide [TTN], a 34 amino acid peptide consisting of residues 17–50. Together, these compounds are sometimes termed endozepines, meaning endogenous BDZ-like substances (although their similarity to BDZs is based on their activity at the BDZ receptor, not their chemical structure). DBI was isolated from rat brain 30 years ago on the basis of its ability to inhibit diazepam binding to the BDZ site on the GABAA receptor (Guidotti et al., 1983). Subsequent studies showed that DBI is not only present in the brains of many species, including humans, but is also widely distributed in peripheral tissues including the liver, kidney, heart, intestine, and various endocrine glands (Bovolin et al., 1990; Costa and Guidotti, 1991; Ferrero et al., 1986a). Within the brain, DBI is synthesized and released mainly from astrocytes (Loomis et al., 2010; Yanase et al., 2002), although a few studies have also reported some expression in nerve cells.
Several early studies investigated the behavioral effects of DBI and ODN using the Vogel conflict test. This test involves training thirsty rats to drink water by licking a metal sipper tube and then giving them a mild shock through the tube. The degree of lick suppression is taken as a measure of the conflict (anxiety) produced by the electric shock. BDZs produce an anticonflict effect that is seen as an increased amount of postshock licking (compared to untreated animals), presumably due to drug-induced enhancement of GABAA receptor function. In contrast, because BDZ inverse agonists reduce GABAA receptor activity, they produce a proconflict effect, manifested by decreased licking compared to control animals. Interestingly, when either DBI or ODN was administered directly into the brains of rats, a proconflict effect was observed (Guidotti et al., 1983; Ferrero et al., 1986b) (see Figure 1). Moreover, in both cases this effect was blocked by treatment with flumazenil, a BDZ receptor antagonist. These findings indicate that DBI and ODN act as inverse agonists at the BDZ receptor in this behavioral test.
Figure 1 Diazepam binding inhibitor has a proconflict effect in rats. Drinking behavior by water-deprived rats was measured under conditions in which a 0.25-mA shock was delivered through the drinking tube. Intracerebroventricular administration of 100 μg of DBI reduced the amount of punished drinking, an effect that was largely blocked by the BDZ receptor antagonist flumazenil (1 mg/kg IV). (After Guidotti et al., 1983.)
There is growing evidence that DBI is broken down into ODN or TTN after its release in the brain, and consequently that these smaller peptides (particularly ODN) are the primary behaviorally active substances in vivo. The initial studies summarized above suggested a role for endozepine peptides in the regulation of anxiety. Subsequent studies have implicated DBI and/or ODN in a wide range of other behavioral processes, including feeding (de Mateos-Verchere et al., 2001), pain perception (Wang et al., 2002), stress responses (Katsura et al., 2002), fear conditioning (Sherrin et al., 2009), and drug addiction (Liu et al., 2005; Ohkuma et al., 2001). It seems likely that at least some of these effects are mediated through endozepine action at the BDZ receptor, although it is important to note that ODN has also been reported to activate a G protein–coupled metabotropic receptor in astrocytes that may be involved in the peptide's inhibitory effect on food intake (do Rego et al., 2007; Hamdi et al., 2012).
Although the reported behavioral effects of DBI and the smaller DBI-derived peptides are intriguing, by themselves they fall short of confirming that these endozepines are truly endogenous ligands for the BDZ receptor. However, two subsequent studies by Christian and coworkers have generated significant support for this hypothesis (Christian and Huguenard, 2013; Christian et al., 2013). The two studies focused on the reticular nucleus of the thalamus (nRT), a structure that plays a key role in regulating information transfer from the thalamus to the cortex via the thalamocortical projections. This regulatory function is mediated by GABAergic inhibition of the thalamic relay neurons, and dysregulation or blocking of GABAergic activity within the nRT causes abnormal electrical activity within the thalamus that can lead to absence seizures (seizures characterized by a brief loss of consciousness but lacking the powerful muscle contractions seen in grand mal seizures). The work of Christian and colleagues yielded the following results: (1) mutant mice lacking the gene that codes for DBI were more susceptible to developing (absence) seizure-like electrical discharges, (2) similar seizure susceptibility was observed in mice in which α3 subunit-containing GABAA receptors were disrupted (most GABAA receptors within the nRT contain this subunit), (3) replacing the DBI gene just in the nRT reversed the effect of the gene loss in the mutant mice, and (4) astrocytes are the likely source of DBI modulation of GABAergic activity within the nRT. Particularly surprising is the finding that in this model system, DBI acts as a positive allosteric modulator (i.e., an agonist, not an inverse agonist) of GABAA receptor activity. The discussion provided in the paper by Christian et al. (2013) offers several theories as to why inverse agonist (negative allosteric modulator) effects have been reported in other studies compared to theirs.
In summary, DBI peptides continue to be the strongest candidates for the long sought-after endogenous ligand(s) for the BDZ receptor. Studies of DBI activity within the nRT are currently the best in showing that endogenous synthesis and release of these peptides serves an important functional purpose. Indeed, the demonstrated regulation by DBI of thalamic electrophysiological activity and its role in suppressing seizure generation suggests that this peptide system could be a future target in the development of anti-epileptic drugs. Given the numerous studies cited earlier that found behavioral (particularly anxiety-related) effects of exogenously administered DBI, more research is needed to whether endogenous DBI plays a role in behavioral regulation and, if so, where in the brain this occurs.
References
Bovolin, P., Schlichting, J., Miyata, M., Ferrarese, C., Guidotti, A., and Alho, H. (1990). Distribution and characterization of diazepam binding inhibitor (DBI) in peripheral tissues of rat. Regul. Pept., 29, 267–281.
Christian, C. A., Herbert, A. G., Holt, R. L., Peng, K., Sherwood, K. D., Pangratz-Fuehrer, S., et al. (2013). Endogenous positive allosteric modulation of GABAA receptors by Diazepam binding inhibitor. Neuron, 78, 1063-1074.
Christian, C. A., and Huguenard, J. R. (2013). Astrocytes potentiate GABAergic transmission in the thalamic reticular nucleus via endozepine signaling. Proc. Natl. Acad. Sci. USA, 110, 20278-20283.
Costa, E., and Guidotti A. (1991). Diazepam binding inhibitor (DBI): A peptide with multiple biological actions. Life Sci., 49, 325–344.
de Mateos-Verchere, J. G., Leprince, J., Tonon, M.-C., Vaudry, H., and Costentin, J. (2001). The octadecaneuropeptide [diazepam-binding inhibitor (33-50)] exerts potent anorexigenic effects in rodents. Eur. J. Pharmacol., 414, 225–231.
do Rego, J.-C., Orta, M.-H., Leprince, J., Tonon, M.-C., Vaudry, H., and Costentin, J. (2007). Pharmacological characterization of the receptor mediating the anorexigenic action of the octadecaneuropeptide: Evidence for an endozepinergic tone regulating food intake. Neuropsychopharmacology, 32, 1641–1648.
Farzampour, Z., Reimer, R. J., and Huguenard, J. (2015). Endozepines. Adv. Pharmacol., 72, 147-164.
Ferrero, P., Conti-Tronconi, B., and Guidotti, A. (1986a). DBI, an anxiogenic neuropeptide found in human brain. In GABAergic Transmission and Anxiety. (G. Biggio and E. Costa, Eds.), pp. 177–185. New York: Raven Press.
Ferrero, P., Santi, M. R., Conti-Tronconi, B., Costa, E., and Guidotti, A. (1986b). Study of an octadecaneuropeptide derived from diazepam binding inhibitor (DBI): Biological activity and presence in rat brain. Proc. Natl. Acad. Sci. U.S.A., 83, 827–831
Guidotti, A., Forchetti, C. M., Corda, M. G., Konkel, D., Bennett, C. D., and Costa, E. (1983). Isolation, characterization, and purification to homogeneity of an endogenous polypeptide with agonistic action on benzodiazepine receptors. Proc. Natl. Acad. Sci. U.S.A., 11, 3531–3535.
Hamdi, Y., Kaddour, H., Vaudry, D., Douiri, S., Bahdoudi, S., Leprince, J., et al. (2012). The stimulatory effect of the octadecaneuropeptide ODN on astroglial antioxidant enzyme systems is mediated through a GPCR. Front. Endocrinol., 3:138. doi:10.3389/fendo.2012.00138.
Iversen, L. (1977). Anti-anxiety receptors in the brain? Nature, 266, 678.
Katsura, M., Mohri, Y., Shuto, K., Tsujimura, A., Ukai, M., and Ohkuma, S. (2002). Psychological stress, but not physical stress, causes increase in diazepam binding inhibitor (DBI) mRNA expression in mouse brain. Mol. Brain Res., 104, 103–109.
Liu, X., Li, Y., Zhou, L., Chen, H., Su, Z., and Hao, W. (2005). Conditioned place preference associates with the mRNA expression of diazepam binding inhibitor in brain regions of the addicted rat during withdrawal. Mol. Brain Res., 137, 47–54.
Loomis, W. F., Behrens, M. M., Williams, M. E., and Anjard, C. (2010). Pregnenolone sulfate and cortisol induce secretion of acyl-CoA-binding protein and its conversion into endozepines in astrocytes. J. Biol. Chem., 285, 21359–21365.
Ohkuma, S., Katsura, M., and Tsujimura, A. (2001). Alterations in cerebral diazepam binding inhibitor expression in drug dependence. A possible biochemical alteration common to drug dependence. Life Sci., 68, 1215–1222.
Sherrin, T., Blank, T., Saravana, R., Rayner, M., Spiess, J., and Todorovic, C. (2009). Region specific gene expression profile in mouse brain after chronic corticotropin releasing factor receptor 1 activation: The novel role for diazepam binding inhibitor in contextual fear conditioning. Neuroscience, 162, 14–22.
Wang, W., Wu, D.-C., Chen, Y.-H., He, W., and Yu, L.-C. (2002). Anti-nociceptive effects of diazepam binding inhibitor in the central nervous system of rats. Brain Res., 956, 393–397.
Yanase, H., Shimizu, H., Yamada, K., and Iwanaga, T. (2002). Cellular localization of the diazepam binding inhibitor in glial cells with special reference to its coexistence with brain-type fatty acid binding protein. Arch. Histol. Cytol., 65, 27–35.