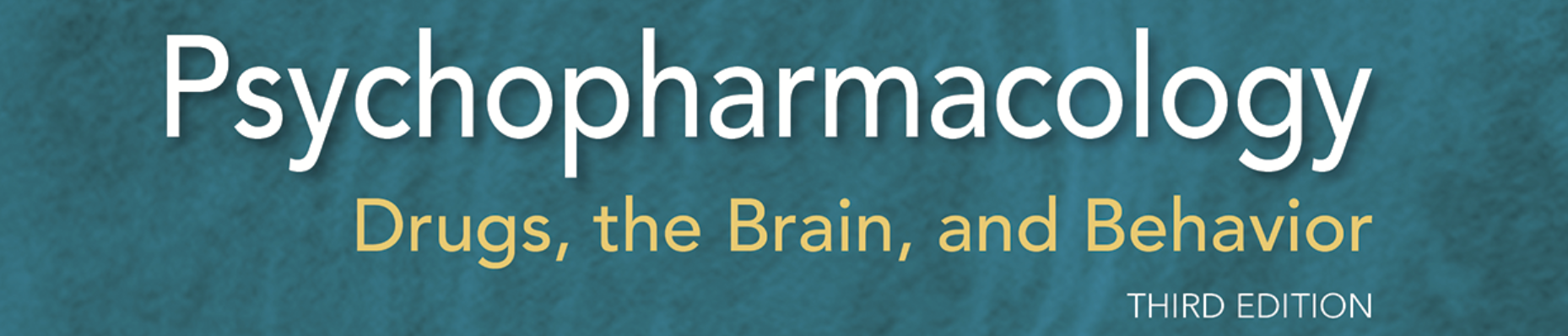
Psychopharmacology 3e Chapter 5 Summary
Catecholamines
Catecholamine Synthesis, Release, and Inactivation
- The major catecholamine transmitters in the brain are DA and NE.
- Catecholamines are synthesized in several steps from the amino acid tyrosine. The first, and also rate-limiting, step in this biochemical pathway is catalyzed by the enzyme TH.
- Catecholamine synthesis can be enhanced by administration of biochemical precursors such as tyrosine and l-DOPA, the latter of which is the main therapeutic agent used in the treatment of Parkinson’s disease.
- Once they have been synthesized, catecholamines are stored in synaptic vesicles for subsequent release.
- Catecholamine release is controlled, in part, by the rate of cell firing. For example, dopaminergic neurons can fire either in single-spike or burst mode, leading to tonic or phasic release of DA. Burst firing causes especially large amounts of DA to be available to postsynaptic cells, primarily because the rate of DA release is greater than the rate at which the neurotransmitter can be cleared and/or metabolized.
- The process of release is controlled by inhibitory autoreceptors located on the cell body, dendrites, and terminals of catecholamine neurons. Terminal autoreceptors function by inhibiting voltage-gated Ca2+ channels and enhancing voltage-gated K+ channels in the terminal membrane.
- DA autoreceptors are of the D2 subtype, whereas NE autoreceptors are of the α2 subtype.
- Catecholamines are inactivated by reuptake from the extracellular fluid mediated by specific DA and NE transporters, and also by enzymatic degradation. MAO-A, MAO-B, and COMT are enzymes that are important in catecholamine metabolism.
- The major catecholamine metabolites are HVA for DA, and MHPG and VMA for NE.
- A number of drugs can modify catecholaminergic function by acting on the processes of synthesis, release, reuptake, or metabolism. Some of these compounds are used either clinically to treat various disorders (e.g., depression, ADHD, and Parkinson’s disease) or experimentally to study the DA or NE system.
Organization and Function of the Dopaminergic System
- The dopaminergic neurons of greatest interest to neuropsychopharmacologists are found near the base of the midbrain in the substantia nigra (A9 cell group) and the VTA (A10 cell group).
- Neurons in the substantia nigra send their axons to the dorsal striatum, thus forming the nigrostriatal tract. The dorsal striatum is composed of two separate structures, called the caudate and putamen, in humans and non-human primates, whereas these structures are fused in rodents.
- The nigrostriatal pathway plays an important role in the control of movement and is severely damaged in Parkinson’s disease. Two different animal models have not only confirmed the involvement of nigrostriatal DA in the initiation of voluntary motor activity (e.g., locomotion), but also implicated this pathway in the regulation of motivation (e.g., motivation to eat and drink) and in cognitive function. These animal models involve either biochemical lesioning of the nigrostriatal tract (e.g., using the neurotoxin 6-OHDA) or the genetic engineering of DD mice.
- Dopaminergic neurons in the VTA form two major dopaminergic systems: the mesolimbic system, which has terminations in several limbic system structures (e.g., nucleus accumbens, septum, amygdala, hippocampus), and the mesocortical system, which terminates in the cerebral cortex (particularly the PFC). The nucleus accumbens is a major constituent of the ventral striatum.
- The mesolimbic and mesocortical DA systems have been implicated in mechanisms of drug abuse, as well as in schizophrenia. A specific subset of VTA dopaminergic neurons activated by rewarding stimuli receive input from the laterodorsal tegmentum and project to the nucleus accumbens, whereas a separate subset that is activated by aversive stimuli receive input from the lateral habenula and project to the medial PFC.
- Researchers have identified five main DA receptor subtypes, designated D1 to D5, all of which are metabotropic receptors. These subtypes fall into two families, the first consisting of D1 and D5, and the second consisting of D2, D3, and D4. The most common subtypes are D1 and D2, both of which are found in large numbers in the striatum and the nucleus accumbens.
- D1 and D2 receptor subtypes can be differentiated partly on the basis that D1 receptors stimulate adenylyl cyclase, thus increasing the rate of cAMP synthesis, whereas D2 receptors decrease the rate of cAMP synthesis by inhibiting adenylyl cyclase. Activation of D2 receptors can also enhance the opening of K+ channels in the cell membrane, which hyperpolarizes the membrane and therefore reduces cell excitability. In addition, D2 receptors have a greater affinity for DA than D1 receptors, thereby leading to the hypothesis that tonic DA release primarily activates D2 receptors, whereas significant activation of D1 receptors requires the additional amount of DA released by phasic activity of the dopaminergic neurons.
- Some of the drugs that affect the dopaminergic system, including DA receptor agonists and antagonists, are presented in Table 5.1. In general, enhancement of dopaminergic function has an activating effect on behavior, whereas interference with DA causes suppression of normal behaviors, ranging from temporary sedation and catalepsy to the profound deficits observed after treatment with a DA neurotoxin such as 6-OHDA.
- When D2 receptor transmission is persistently impaired either by chronic antagonist administration or by denervation (e.g., 6-OHDA lesions), animals become supersensitive to treatment with a D2 agonist. This response is mediated at least in part by up-regulation of D2 receptors by postsynaptic neurons in areas such as the striatum.
Organization and Function of the Noradrenergic System
- The most important cluster of noradrenergic neurons is the A6 cell group, which is located in a region known as the locus coeruleus. These neurons innervate almost all areas of the forebrain and mediate many of the important behavioral functions of NE.
- NE and EPI released into the bloodstream as hormones work together with NE released from sympathetic neurons to mediate the physiological reactions that make up the “fight-or-flight” response. These reactions include (1) vascular changes that direct more blood flow to skeletal muscles, heart, and brain; (2) increases in blood pressure, heart rate, and cardiac muscle contractile force; (3) respiratory changes that produce increased oxygen intake; (4) reduced digestive activity; (5) pupil dilation; (6) increased availability of metabolic fuels due to liberation of sugar from the liver and fats from adipose tissue; and (7) decreased secretion of gastric juices and saliva.
- NE and EPI activate a group of metabotropic receptors called adrenoceptors. They are divided into two broad families, α and β, which are further subdivided into α1, α2, β1, and β2. Both β-receptor subtypes enhance the synthesis of cAMP, whereas α2-receptors inhibit cAMP formation.
- Another mechanism of action of α2-receptors involves hyperpolarization of the cell membrane by stimulating K+ channel opening. In contrast, α1-receptors increase the intracellular concentration of Ca2+ ions by means of the phosphoinositide second-messenger system.
- The noradrenergic system is involved in many behavioral functions, including arousal, cognition, and the consolidation of emotional memories (e.g., related to fear-inducing situations).
- The arousal- and wakefulness-promoting effects of NE are primarily mediated by pathways from the LC to the medial septal, medial preoptic, and lateral hypothalamic areas. Both α1- and β-receptors are involved in these effects. LC neurons constitute one of the components of an arousal circuit that also includes orexin cells of the lateral hypothalamic area.
- The cognitive-enhancing effects of NE (e.g., on attention and working memory) are mediated by activation of high-affinity α2-adrenoceptors in the PFC. Activation of lower-affinity α1-receptors has the opposite effect, namely a decrease in cognitive function. Consequently, the relationship between NE release in the PFC and cognition can be described as an inverted U-shaped function: optimum cognitive function occurs at moderate amounts of NE release, whereas either too little or too much release can cause cognitive impairment.
- The facilitatory role of α2-adrenoceptors in cognition has led to the use of the α2-agonist guanfacine for the treatment of ADHD and Tourette’s syndrome.
- Both central NE and peripherally released EPI play roles in memory consolidation. The mechanism by which EPI promotes memory is still being studied, with one theory focusing on activation of β-receptors on the vagus nerve and a competing theory focusing on a role for EPI-mediated elevations in plasma glucose. Administration of glucose has been shown to mimic the facilitatory effects of EPI in some studies, including studies of memory enhancement in aged animals.
- Adrenergic agonists are used therapeutically for various physiological and psychological disorders. These include the α1-agonist phenylephrine, which helps relieve nasal congestion; the α2-agonist clonidine, which is used in the treatment of hypertension and drug withdrawal symptoms; and β2-agonists such as albuterol, which is an important medication for relieving bronchial congestion in people suffering from asthma.