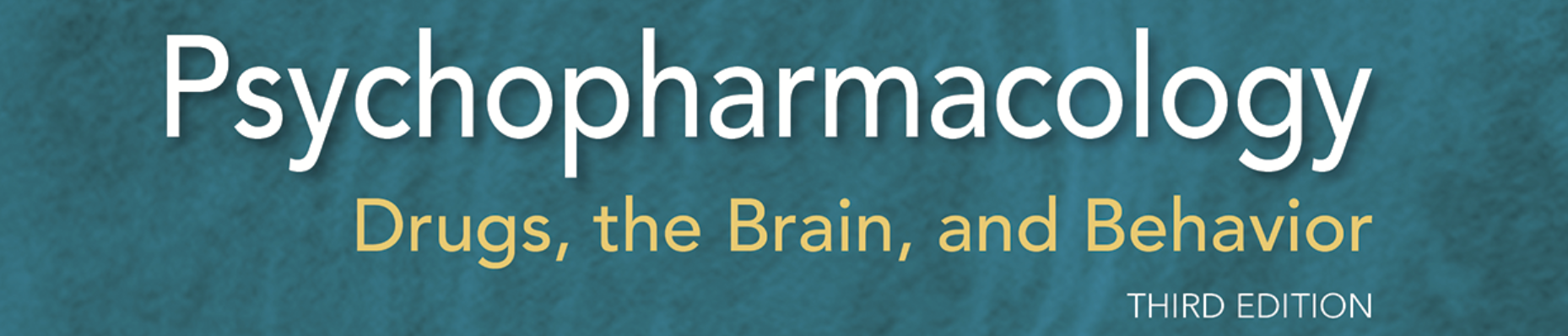
Psychopharmacology 3e Chapter 2 Summary
Structure and Function of the Nervous System
Cells of the Nervous System
- Neurons are surrounded by a cell membrane and are filled with cytoplasm and the organelles needed for optimal functioning.
- Among the most important organelles are the mitochondria, which provide energy for the metabolic work of the cell.
- The principal external features of a neuron are the soma, treelike dendrites, and a single axon extending from the soma that carries the electrical signal all the way to the axon terminals.
- Axon terminals contain synaptic vesicles filled with neurotransmitter molecules that are released into the synapse between cells when the action potential arrives.
- The dendrites of a neuron are covered with minute spines that increase the receiving surface area of the cell. These spines are reduced in size in individuals with intellectual impairment and reduced in number in those with schizophrenia.
- Thousands of receptors that respond to neurotransmitters released by other neurons are found on the dendrites, dendritic spines, and soma of the cell.
- The axon hillock is located at the juncture of soma and axon and is responsible for summation (or integration) of the multiple signals required to generate an action potential.
- Conduction of the action potential along the axon is enhanced by the insulating property of the myelin created by nearby glial cells.
- The nucleus of the cell is located within the soma, and protein synthesis occurs there. Transcription of the genetic code for a specific protein by mRNA occurs within the nucleus, and translation of the “recipe,” carried by the mRNA, occurs on the ribosomes in the cytoplasm. Ribosomes link together appropriate amino acids to create the protein.
- Changes in synaptic activity increase or decrease the production of particular proteins by activating transcription factors in the nucleus.
- Epigenetics is the study of how environmental demands such as diet, environmental toxins, stress, prenatal nutrition, and many others turn on or turn off the expression of specific genes. Although epigenetic markers do not modify DNA, they can last a lifetime and may be transmitted to future generations. Two common markers are DNA methylation and chromatin remodeling.
- Future drug development will target epigenetic factors to treat psychiatric disorders that have genetic components by turning on protective genes or turning off genes associated with symptom development.
- Newly manufactured proteins are packaged into vesicles in the soma and are moved by motor proteins that slide along the neuron’s microtubules (part of the cytoskeleton) to the terminals (anterograde transport). Protein waste and cell debris are transported from the terminals back to the soma (retrograde transport) for recycling.
- The cell membrane is a phospholipid bilayer that prevents most materials from passing through, unless the material is lipid soluble. Special transporters carry other essential materials, such as glucose, amino acids, and neurotransmitters into the cell. Ion channels allow ions such as Na+, K+, Cl–, and Ca2+ to move across the membrane. Other proteins associated with the membrane include receptors and enzymes.
- Four types of glial cells are found in the nervous system. Schwann cells and oligodendroglia produce the myelin sheath on peripheral and central nervous system neurons, respectively. Astrocytes regulate the extracellular environment of the neurons, regulate CNS blood flow, and provide physical support and nutritional assistance. Microglia act as phagocytes to remove cellular debris and provide immune function.
Electrical Transmission within a Neuron
- At rest, neurons have an electrical charge across the membrane of –70 mV (resting potential), with the inside being more negative than the outside.
- The resting potential results from the balance between two competing forces on K+ ions. Electrostatic pressure moves K+ inward because it is attracted by negatively charged molecules trapped inside the cell. The concentration gradient for K+ pushes ions out of the cell in an effort to distribute them evenly.
- The Na+–K+ pump also helps to maintain the negative membrane potential by exchanging three Na+ ions (moved out of the cell) for two K+ ions (taken in).
- Local potentials are small, short-lived changes in membrane potential found largely on the soma and dendrites after the opening of ligand-gated channels.
- Excitatory postsynaptic potentials (EPSPs), or depolarizations, occur when ligand-gated Na+ channels open and allow Na+ to enter the cell on its concentration gradient, making the cell slightly more positive and bringing the membrane potential closer to the threshold for firing. Opening Cl– channels allows Cl– to enter on its concentration gradient, making the cell more negative and farther from the threshold, causing hyperpolarizations called inhibitory postsynaptic potentials (IPSPs). When ligand-gated K+ channels open, K+ exits on its concentration gradient, leaving the cell more negative inside and farther from the threshold producing an IPSP.
- The summation of all EPSPs and IPSPs occurring at any single moment in time occurs at the axon hillock. If the threshold (–50 mV) is reached, voltage-gated Na+ channels open, allowing large amounts of Na+ to enter the cell to produce the massive depolarization known as the action potential.
- At the peak of the action potential (+40 mV), voltage-gated Na+ channels close and cannot be opened until they reset at the resting potential, so no action potential can occur during this time (the absolute refractory period).
- As the cell becomes more positive inside, voltage-gated K+ channels open and K+ exits from the cell, bringing the membrane potential back toward resting levels. The overshoot by K+ causes the cell to be more polarized than normal, so it is more difficult to reach the threshold to generate another action potential (relative refractory period).
- The action potential moves down the length of the axon by sequential opening of voltage-gated Na+ channels.
- In myelinated axons, regeneration of the action potential occurs only at the nodes of Ranvier, producing a rapid, saltatory conduction that is more energy efficient because the Na+–K+ pump needs to exchange ions only at the nodes.
- The characteristics of local and action potentials are summarized in Table 2.2.
Organization of the Nervous System
- The central nervous system (CNS) includes the brain and the spinal cord. The remaining nerves of the body constitute the peripheral nervous system (PNS).
- The PNS is divided into the somatic nervous system, which includes spinal nerves that transmit sensory and motor information for skeletal muscles, and the autonomic nervous system, which serves smooth muscles, glands, and visceral organs.
- The autonomic nervous system has two divisions: the sympathetic, which serves to mobilize energy for times of “fight-or-flight”; and the parasympathetic, which reduces energy usage and stores reserves.
- The CNS is protected by a bony covering, three layers of meninges (dura, arachnoid, and pia), and cerebrospinal fluid.
- Neurotrophic factors are neuronal growth factors that guide the development of neurons, regulate dendritic growth and retraction, and aid in survival of neurons.
- The CNS can be divided into six regions containing multiple nuclei and their associated axons, which form interconnecting neural circuits: spinal cord, myelencephalon, metencephalon, mesencephalon, diencephalon, and telencephalon.
- The gray matter of the spinal cord constitutes cell bodies that receive sensory information and cell bodies of motor neurons that serve muscles. The white matter consists of tracts of myelinated axons that carry signals in the ascending direction, to the brain, and the descending direction, for cortical control of the spinal cord.
- Continuous with the spinal cord is the myelencephalon, or medulla, which contains nuclei that serve vital functions for survival, such as respiration, heart rate, and vomiting.
- The metencephalon includes two major structures. The cerebellum functions to maintain posture and balance and provides fine motor control and coordination. The pons contains several nuclei that represent the origins of most of the tracts utilizing the neurotransmitters norepinephrine (the locus coeruleus) and serotonin (the raphe nuclei).
- Beginning in the medulla, running through the pons, and extending into the midbrain is the reticular formation—a network of interconnected nuclei that control arousal, attention, and survival functions.
- The mesencephalon, or midbrain, contains nuclei that control sensory reflexes such as pupillary constriction. Other nuclei (substantia nigra and ventral tegmental area) are the cell bodies of neurons that form three major dopaminergic tracts. The periaqueductal gray organizes behaviors such as defensive rage and predation and serves as an important pain-modulating center.
- The diencephalon contains the thalamus, which relays information to the cerebral cortex, and the hypothalamus, which is important for maintaining homeostasis of physiological functions and for modulating motivated behaviors, including eating, aggression, reproduction, and so forth. The many nuclei that constitute the hypothalamus control both the autonomic nervous system and the endocrine system.
- The telencephalon includes both the cerebral cortex and multiple subcortical structures, including the basal ganglia and the limbic system. The basal ganglia modulate movement.
- The limbic system is made up of several brain structures with perfuse interconnections that modulate emotion, motivation, and learning. Some of the prominent limbic structures are the amygdala, hippocampus, nucleus accumbens, and limbic cortex.
- The six-layered cerebral cortex is organized into four lobes: the occipital, temporal, and parietal, which are the sensory lobes involved in perception and memories, and the frontal, which regulates motor movements and contains the “executive mechanism” for planning, evaluating, and making strategies.
- Although there are differences in absolute and relative size, rat and human brains have the same major subdivisions, which are topographically organized in similar locations. Rat brains have relatively larger olfactory bulbs and midbrains. Human brains have expanded secondary and tertiary association areas of the cerebral cortex that serve higher-order sensory perception and cognitive functions.